Research
Current projects
Solenemar [FP7-CAPACITIES, project #229390]
"Strenghtening the SOLid-state research capacities in Zagreb by the introduction of Nuclear Magnetic Resonance method"
Given that nuclear magnetic resonance (NMR) is today an unavoidable method in solid-state physics, we aim this project at complementing the infrastructure for materials research at the Physics Department – Faculty of Science (PDFS) by the introduction of a solid state NMR (ssNMR). With this project, we shall accomplish four tasks: (a) strengthen the human potential in solid state research, (b) purchase and install an ssNMR spectrometer, (c) further develop a cooperation with European NMR centres of excellence, and (d) make the overall research capabilities of PDFS and especially ssNMR method visible to the wider scientific community, general public, and small and medium enterprises (SME) in Croatia and South Eastern Europe (SEE). Human potential will be strengthened by: (i) an intensive training of young researchers in high-quality European NMR laboratories, (ii) the return of experienced researchers from abroad, (iii) the exchange of know-how and experience by secondments of senior Croatian researchers and top European NMR researchers. The promotion of PDFS will be achieved by: (i) the organisation of two workshops with targeted audience of young researchers in SEE, (ii) the organisation of seminars for industry and SME managers about the possibilities of materials research at PDFS, (iii) active participation of Croatian researchers in EUROMAR conferences and European magnetic resonance societies, and (iv) the interaction with the public media. The new ssNMR group will reinforce the overall European research area since the need for NMR investigations of new materials highly exceeds the European experimental ssNMR capacities.(more)
Microwave investigations of new materials [MZOS project #119-1191458-1022]
With the development of new material fabrication technologies and with the miniaturization of devices, the physical properties of materials are increasingly dependent on their size and surface effects. This is especially important if the characteristic length scales of the corresponding bulk material are larger than that of one, two, or all three dimensions of the investigated sample. In that case, the new sample has reduced dimensionality (2D, 1D or 0D). This project will focus on the electric and magnetic properties of reduced dimensionality systems, probed by microwave radiation (9-20 GHz). Special scrutiny will be given to high- and low-temperature superconductors in nanometer-range thickness, fullerenes and their derivatives, and carbon nanotubes.
High Temperature Superconductors
High-temperature superconductors (HTS) are materials that have a superconducting transition temperature (Tc) above 30 K. Until the discovery of Fe-based pnictide superconductors in 2008, the term HTS was used as a synonym for cuprate based superconductors. The first HTS La2-xBaxCuO4 (Tc = 38 K) was discovered in 1986 by K. A. Müller and J. G. Bednorz, for which they were awarded the Nobel Prize in Physics in 1987. Since then other HTS compounds were found, e.g. YBa2Cu3O7 (Tc = 92 K), HgBa2Ca2Cu3O8 (Tc = 135 K), etc. Although the discovery of HTS dates back 20 years, there is no widely accepted theory to explain their properties.
The crystal structure of all HTS consists of two dimensional copper oxide planes that are separated by other atoms like Sr, La, Y or Ba, and some additional oxygen atoms. The atoms in between the layers serve as dopands. For example if one dopes La2CuO4 with Sr, one introduces charge carriers (holes) into the CuO2 planes. Only by doping, the crystal becomes superconducting.
Simplified doping dependent phase diagram of cuprate superconductors consists of the antiferromagnetic (AF) phase close to zero doping, the superconducting phase around optimal doping, and the pseudogap phase. A pseudogap is a term which describes an energy (normally near the Fermi energy) which has very few states associated with it. One of the first observations was in NMR measurements of YBa2Cu3O6+x by Alloul et al.[1] The origin of the pseudogap is controversial and still subject to debate in the condensed matter community. Two main interpretations are emerging: 1. The scenario of preformed pairs, 2. The scenario of a non superconducting related pseudogap.
Nuclear magnetic resonance (NMR)
NMR is powerful, nonagressive experimental technique, based on magnetism of atomic nuclei. The method is famous for it's application in medical diagnostics, but it has much broader field of applications. In material sciences its main application is in structural investigations. NMR is capable to precisely determine the existence, postition and coordination of atoms in complicated molecules or crystals.
Discovered by physicists, the first application of the NMR method was in condensed matter physics, and it remains one of the most useful methods for investigations of interactions in solid state. NMR is direct, nonperturbative microscopic probe for magnetism which i) give precise static and dynamic information about localized or itinerant electrons; ii) reveals eventual inhomogeneity due to impurities or charge/spin density waves; iii) discovers existence and type of phase transitions. NMR has proven its strength in investigations of HTS, strongly correlated electronic systems (heavy fermions, quantum magnetism of spin systems), and all other systems in solid state physics
NMR uses the nucleus as a local probe. The nucleus possesses a magnetic moment and an angular momentum, called spin. The nuclei of atoms like 1H or 13C possess a spin of I=1/2, the nuclei of other atoms can have higher spins, e.g. 17O: I=5/2, 63Cu: I=3/2. Whenever I > 1/2, the nucleus possesses also a quadrupole moment. Thus the spin of nuclei can interact with the surrounding electronic spins, i.e. probe magnetic interactions, and the quadrupole moment can interact with the surrounding electric field gradient, i.e. probe electric interactions. One can compare the spin of the nucleus with a magnetic dipole that rotates around its own axis. In an external magnetic field B the dipole alignes with the lines of magnetic flux and starts to precess like a whipping top around them. The precession frequency is called Larmor frequency ω0, which is proportional to the external magnetic field B: ω0 = &gama;B, where &gama; is called gyromagnetic ratio. In magnetic field of 12 T, the Larmor frequecy of 1H atoms amounts 510.89 MHz, while for e.g. 63Cu it amounts 135.41 MHz. By applying radio frequency of the same frequency ?0 to the nucleus, the spin of the nucleus flips. One can apply this radio frequency by applying short pulses of radio frequency to flip the spin for a certain angle, e.g. 90°. After a pulse of radio frequency the spin relaxes back to its equilibrium position, i.e. it alignes with the external magnetic field again. While the nucleus relaxes back, it emits radio frequency itself. This radio frequency can be measured. The radio frequency that is emitted by the nucleus after the pulse has slightly different frequencies dependeing on where the nucleus is located in the compound, i.e. dependeing on the magnetic and electronic surrounding of the nucleus. Thus, the nuclei act as alittle spies that reveal local charge distribution and interactions in the studied material.
Microwave response of materials
Microwaves are electromagnetic waves with frequencies between 1 GHz and 1 THz (higher than radio frequencies and lower than infrared). In experimental solid state physics, the microwave investigations are useful to study high-frequency transport properties. Frequencies are high enough to avoid the application of electrical contacts, but still low enough to avoid excitations of phonons or e.g. quasiparticles in superconductors.
There are several possible experimental arrangements for the measurements of microwave response of a sample of interest. We use the method of perturbation of a resonant microwave cavity. Microwave cavity is box made of very conducting material whose dimensions are comparable to the wavelength (~3 cm). When in resonance, standing electromagnetic wave is formed in the cavity. When a sample is placed into the cavity, it causes a weak perturbation to the cavity configuration. The resonant frequency and the Q-factor of the cavity are slightly changed. These changes can be measured, and one obtains valuable information about conductivity, permitivity and/or permeability of the studied sample.
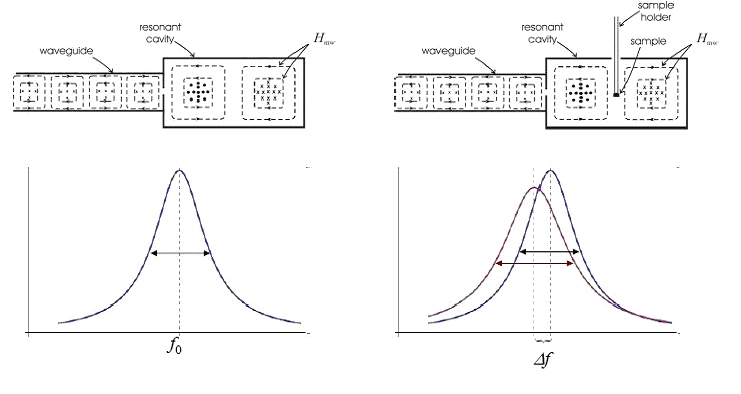
Our microwave cavity
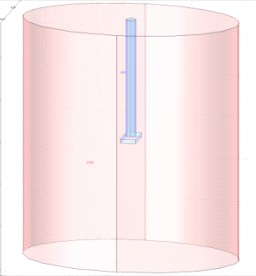
Propagation of an electromagnetic wave into a medium
Wave propagation is determined by wave vector k. In vacuum, wave vector is a real quantity given by
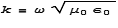

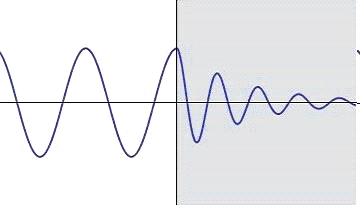

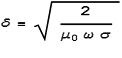
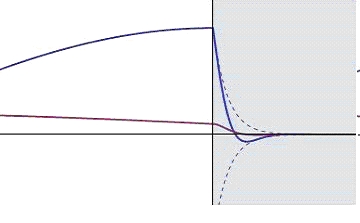
At very low temperatures some materials become superconducting. The conductivity becomes purely imaginary (current delays after voltage by 90 degrees):
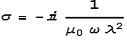

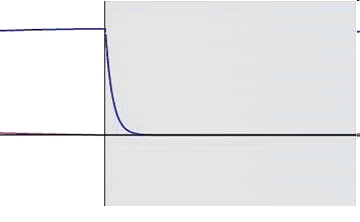
Surface impedance
The two measured quantities (Q-factor and frequency shift) can be combined into one complex quantity – complex frequency shift:
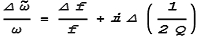
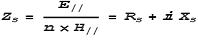

![]() |
![]() |
Recent publications
- M.S. Grbić, N. Barišić, A. Dulčić, I. Kupčić, Y. Li, X. Zhao, G. Yu, M. Dressel, M. Greven, and M. Požek, "Distinctive behavior of superconducting fluctuations and pseudogap in nearly optimally doped single crystal of HgBa2CuO4+?", Physica C 470, S228 (2010)
- M. S. Grbić, N. Barišić, A. Dulčić, I. Kupčić, Y. Li, X. Zhao, G. Yu, M. Dressel, M. Greven, and M. Požek," Microwave measurements of the in-plane and c-axis conductivity in HgBa2CuO4+?: Discriminating between superconducting fluctuations and pseudogap effects", Phys. Rev. B 80, 094511 (2009)
- A. Narduzzo, M. S. Grbić, M. Požek, A. Dulčić, D. Paar, A. Kondrat, C. Hess, I. Hellmann, R. Klingeler, J. Werner, A. Köhler, G. Behr, and B. Büchner," Upper critical field, penetration depth, and depinning frequency of the high-temperature superconductor LaFeAsO0.9F0.1 studied by microwave surface impedance", Phys. Rev. B 78, 012507 (2008)
- M. Požek, I. Kupčić, A. Dulčić, A. Hamzić, D. Paar, M. Basletić, E. Tafra, and G. V. M. Williams ," Microwave and magnetotransport properties of RuSr2RCu2O8 (R=Eu,Gd) doped with Sn", Phys. Rev. B 77, 214514 (2008)
- M. Požek, A. Dulčić, A. Hamzić, M. Basletić, E. Tafra, G.V.M. Williams and S. Krämer, "Magnetotransport of lanthanum doped RuSr2GdCu2O8 - the role of gadolinium", Eur. Phys. J. B 57, 1 (2007)
- M.S. Grbić, D. Janjušević, M. Požek, A. Dulčić and T. Wagner, "Microwave study of magnetic field penetration parallel to thin niobium films", Physica C 460, 1293 (2007)
- D. Janjušević, M. S. Grbić, M. Požek, A. Dulčić, D. Paar, B. Nebendahl, andT. Wagner, "Microwave response of thin niobium films under perpendicular static magnetic fields", Phys. Rev. B 74, 104501 (2006)
Finished projects
Nov. 2000 - Nov. 2001 : MZT project # 00-219 "Remote Control and Analysis of Experiment - DKIA", Informatic project of the Ministry of Science and Technology of Croatia (budget 4.000 EUR) 1997 - 2000 : MZT project # 098439 "Investigation of Microwave Complex Conductivity of Superconductors", Young researchers stimulating project of the Ministry of Science and Technology of Croatia (budget 5.000 EUR)
PARTICIPANT:
2002-2006 : MZOS project #0119275 "Microwave Investigations of Superconductors", Croatian Ministry of Science, Education and Sports; principal investigator prof. dr. sc. Antonije Dulčić 2005 : FP6 project #516938 "WYP2005 EUROPE", European Commission; in charge for Croatian work package: Dr. Slobodan Milošević 1996-2002 : MZT project #119225 "Microwave properties of superconductors", Ministry of science and technology of Croatia; principal investigator prof. dr. sc. Antonije Dulčić 1996-2002 : MZT project # 119201 "Collective phenomena in new materials", Ministry of science and technology of Croatia; principal investigator prof. dr. sc. Amir Hamzić 1996-2002 : MZT project #00980610 "Relaksation processes of ferroelectrics and superconductors", Ministry of science and technology of Croatia; principal investigator Dr. Boris Rakvin, Scientific adviser 1996-1998 : VW project "Mikrowelleneigenschaften von Supraleitern in hohen Magnetfeldern", Volkswagen-foundation; principal investigators Prof. Dr. Michael Mehring and Prof. Dr. Antonije Dulčić 1990-1995 : MZT project #1-03-063 "Magnetskic resonance of superconductors and ferroelectrics", Ministry of science and technology of Croatia; principal investigator prof. dr. sc. Antonije Dulčić