Nils Paar obtained his Ph.D. in theoretical nuclear physics from Technical University Munich in 2003 under the supervision of Prof. Peter Ring. Following a postdoctoral position at Technical University Darmstadt until 2006, he joined the University of Zagreb Faculty of Science, where he is currently serving as a full professor. From 2014-2015 he was a Marie Curie research fellow at Universitaet Basel, Switzerland. His research activities in exotic nuclear structure and dynamics, astrophysically relevant weak-interaction processes and neutrino-induced reactions, resulted in publication record comprising over 137 papers in international scientific journals and conference proceedings, with over 4000 citations (WoS). Over the course of his career, he has overseen and mentored a multitude of scholars, guiding them through Master's, Ph.D., and postdoctoral research programs, in addition to hosting international guests. He has actively participated in international conferences and workshops, delivering more than 67 talks, including 34 invited presentations. Nils Paar served as the head of the Division for Theoretical Physics, the head of the Department of Physics at the Faculty of Science, and as a member of the Senate at the University of Zagreb.
Modern Theory of Nuclear Structure
and Nuclear Astrophysics

The field of nuclear structure theory is rapidly evolving from macroscopic and microscopic models applied to stable nuclei, toward modern density functional theory applied in the uncharted territories of nuclide map encompassing vast regions of short-lived and exotic nuclei away from the valley of beta-stability. Our primary aim is to achieve a comprehensive understanding of nuclei and their excited states, grounded in a fundamental understanding of the underlying nuclear interaction. The precise description of nuclear properties holds paramount importance in astrophysics, influencing critical processes such as stellar evolution and nucleosynthesis, with accurate global microscopic calculations serving as essential tools for astrophysical applications. Within the Eleven Science Questions for the New Century lies a compelling mystery: How were the elements from iron to uranium made? With a good chance of answering this question in the near future, nuclear structure and astrophysics find themselves on the threshold of the most exciting era in decades.
The Properties of Nuclei in Hot Stellar Environment
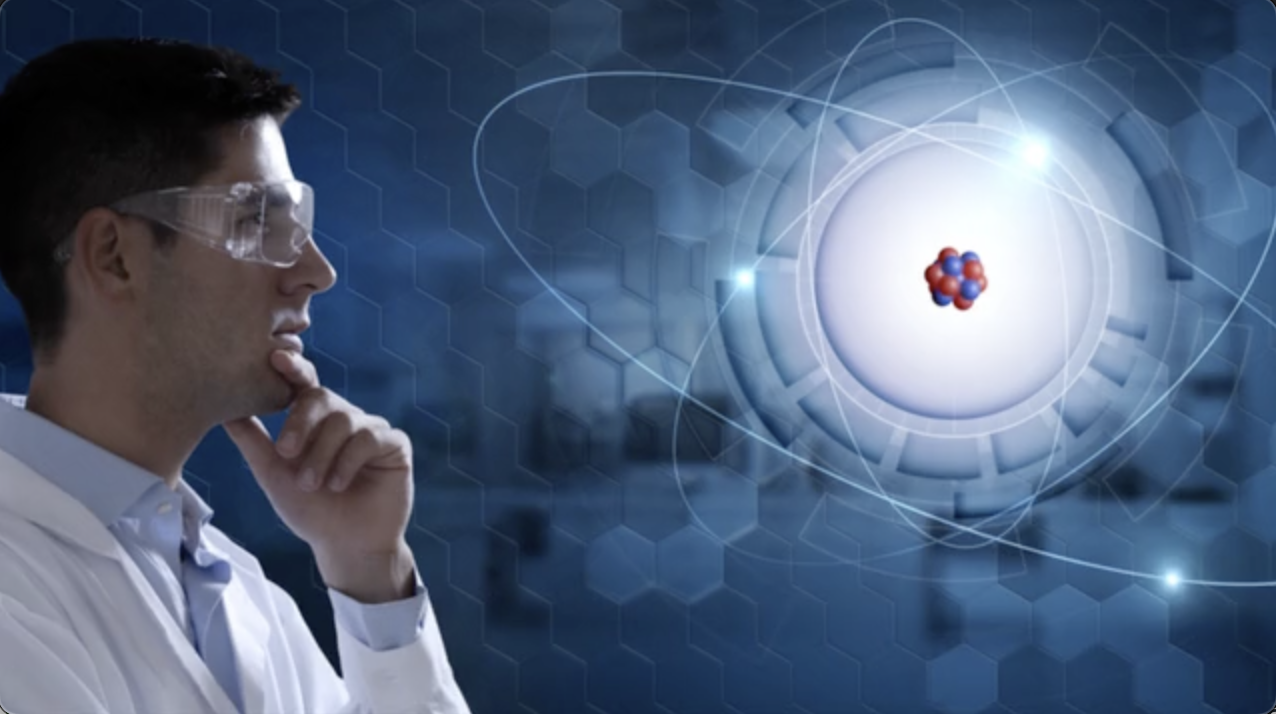
One of the most important challenges in nuclear physics and nuclear astrophysics is the understanding how the nuclear chart and its limits - drip lines - evolve with increasing temperature, considering that nuclei participating in most of the processes in the Universe are hot. Therefore, it is essential to know how many protons and neutrons can be bound together in hot stellar environment. Therefore we aim to understand which nuclei can contribute to nuclear reactions and processes, especially in extremely hot stellar environments such as supernovae and neutron star mergers, where most of the chemical elements heavier than iron are produced. The knowledge about nuclear properties at finite temperature is largely unknown, since most of theoretical and experimental studies are restricted to zero temperature. Only recently, we have established a theory framework based on relativistic nuclear energy density functional that allowed us to map the position of nuclear drip lines at finite temperature for the first time (see Video Byte by Research Square).
Exotic Modes of Nuclear Excitations

The extreme isospin of nuclei far from stability and their weak
binding reveal unique structure phenomena such as neutron
halo
and
skin,
which play a crucial role in understanding of the
nuclear many-body problem
at the
limits of stability.
The multipole response of nuclei far from the beta-stability line and the possible
occurrence of new exotic modes of excitation presents a rapidly
growing field of research.
The properties of
observed low-energy dipole excitations in nuclei with a pronounced neutron
excess is currently very much under discussion due to their unique
properties.
A new excitation mode has been suggested by theory calculations
to appear also in nuclei close to the proton drip-line:
proton pygmy dipole
resonance whose dynamics is governed by vibrations of protons from
weakly bound orbits against the core composed of other nucleons.
This exotic mode is currently awaiting for its
experimental confirmation.
The exotic modes of excitation are interesting not only as new physical phenomena, but also because they could play an important role in astrophysically relevant processes. The description of multipole spin-flip and isospin-flip excitations is essential in calculations of beta-decay, electron capture and neutrino-nucleus interaction rates. The low-energy dipole transition strength in neutron-rich nuclei have a pronounced effect on the calculated r-process abundances, i.e. on the production of chemical elements and on the propagation of ultra-high energy cosmic rays. On the proton-rich side the proton pygmy dipole resonance could contribute to the nucleosynthesis in rapid proton capture processes, as well as in the two-proton capture in astrophysical conditions characteristic for explosive hydrogen burning in novae and x-ray bursts.
Neutrino-Nucleus Reactions and Weak Interaction Rates
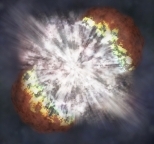
Neutrino-nucleus reactions are of central importance in astrophysics
where the transport of neutrinos determines the rate of cooling of
many stellar objects, and their detection provides a unique way of
looking at such fascinating astrophysical phenomena as the interior
processes in our sun,
supernovae
explosions, and stellar nucleosynthesis.
These reactions are also essentially connected to another of the
Eleven
Science Questions for the New Century: do neutrinos have
mass? Since nuclei are used as
neutrino detectors in solar
experiments, supernova observatories and in
neutrino oscillation measurements,
it is of outmost importance to achieve a quantitative description
of neutrino-nucleus reactions in a fully microscopic theory.
Description of a neutrino-nucleus cross section becomes increasingly complicated as the target mass number increases and novel accurate approaches based on relativistic energy density functionals must be developed and applied in calculations for all relevant neutrino-induced reactions. More generally, microscopic nuclear structure theory must be integrated into various astrophysical models of nucleosynthesis , supernova dynamics, and neutrino-induced reactions, by providing accurate global predictions for bulk nuclear properties and nuclear transitions. Understanding of the nucleosynthesis of heavier elements during the r-process necessitates systematic knowledge on the neutrino-nucleus cross sections not only in stable nuclei but also in nuclei away from the valley of beta stability.
The Key Research Methods
Relativistic density functional theory
Utilizing the most advanced energy density functionals, this approach provides a coherent, universally applicable, and quantitative description of nuclei. It allows studies of exotic nuclear structure and dynamics, extrapolations into uncharted territories of the nuclide map, extending towards the particle drip lines.
Theory of Nuclear Weak Interactions
The theory of nuclear weak interactions, formulated within the framework of the electroweak theory, describes the fundamental mechanism responsible for processes such as beta decay and electron capture in atomic nuclei or neutrino - nucleus interaction. It plays a crucial role in understanding the transformation of one type of elementary particle into another within the atomic nucleus.
Advanced Scientific Computing
It serves as an essential method for tackling the intricate challenges posed by the relativistic nuclear many-body problem, e.g., solving coupled systems of differential equations, vast numbers of nuclear matrix elements, and extensive eigenvalue problems. Notably, it is an indispensable method for large-scale calculations across the nuclide chart, contributing significantly to the advancement of nuclear physics research.
More details are available in the listed publications and references therein.